Mom and dad genes and the intricate process of gene regulation
If the genome is like computer hardware, then the epigenome is the software that turns certain genes on and others off. During development, the epigenome mediates the complex process that regulates gene expression by selectively turning genes on and off to lead a cell to become a skin cell or a neuron, for instance.
The epigenome is to a large extent encoded as a set of cell-type specific chemical modifications of DNA called DNA methylations. In his lab at Baylor College of Medicine, Dr. Aleksandar Milosavljevic and his colleagues at the National Institutes of Health Roadmap Epigenomics Project look to better understand cellular mechanisms of gene regulation. In this particular work, they studied a mechanism called sequence-dependent, allele-specific methylation or SD-ASM.
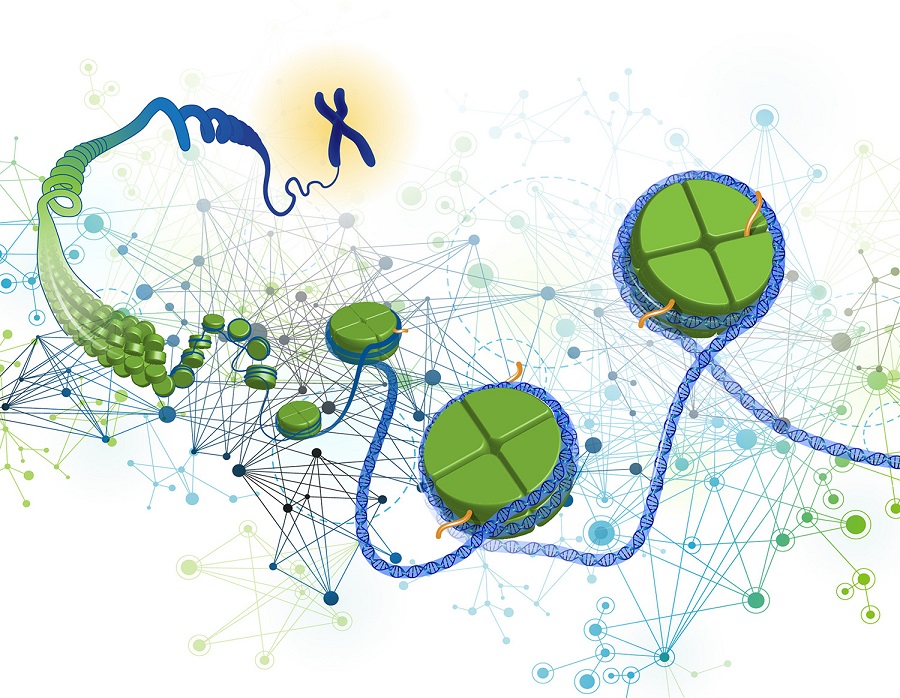
“We used a method called whole-genome bisulfite sequencing (WGBS) that allowed us to see gene regulation with single-molecule resolution across a multitude of human cell types,” said Milosavljevic, professor of molecular and human genetics and co-director of the Computational and Integrative Biomedical Research Center at BCM. “We were able to determine epigenomic differences in the same gene in the paternal and maternal chromosomes in individual cells. We could see things that could not be seen before.”
Digital and stochastic
Using this high-resolution approach, Milosavljevic and his colleagues discovered that SD-ASM-mediated gene regulation works in a way similar to that of a home thermostat, but has unique features. A thermostat has a preset temperature at which the air conditioning machine will turn on. The AC machine will turn off when the temperature is below the preset value. The process is digital, meaning that the AC machine is either on or off. The researchers discovered that, like a home thermostat, this gene regulation mechanism is also digital; it turns a gene either on or off for a fraction of the time and there are no intermediate levels of activity.
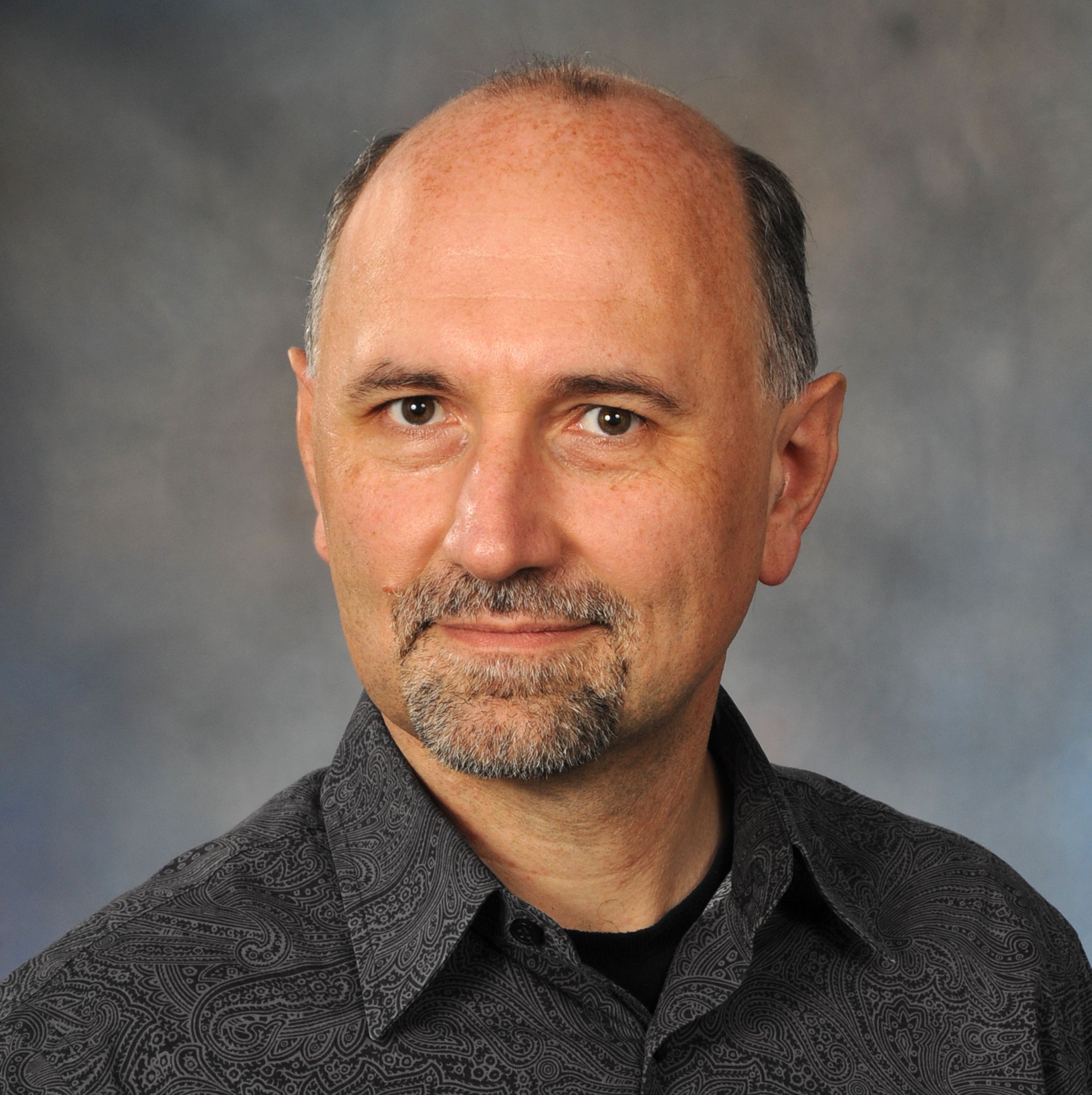
“We also discovered that this process of gene regulation is stochastic,” said Milosavljevic, who also is a member of Baylor’s Dan L Duncan Comprehensive Cancer Center. “Returning to the thermostat analogy, in a regular thermostat, the probability of the AC turning on when the temperature reaches the preset value is 100 percent. In a stochastic thermostat, on the other hand, the probability of the AC machine turning on increases as the temperature reaches the preset value, but is not 100 percent once the temperature is reached. There is still a chance that the AC will not turn on, but the probability of the AC turning on is higher as the temperature raises.”
In the case of the gene regulation mechanism, specific genetic variants that control the paternal and maternal genes may represent different ‘preset temperature values’ in the thermostat analogy, causing differences in the degree maternal and paternal genes are expressed in each human cell.
“For example, for a particular gene in a neuron, the maternal’s genetic ‘thermostat’ may be set to ‘turn on the gene at 73 degrees’, and the paternal at ’74 degrees.’ That means that the paternal and maternal genes are turned on differently and that creates an imbalance between the two copies of that gene,” Milosavljevic said. “We found that between 5 and 8 percent of the epigenome shows this type of imbalance.”
The implications of these findings extend to a number of biomedical fields. For instance, their findings may add another layer of complexity that so far has not been taken into account in certain intricate human diseases.
If we add this layer of complexity, we might be able to better understand how dosage-sensitive genes may contribute to human diseases that have so far been hard to tackle, such as neuropsychiatric disorders,” Milosavljevic said. “We hope this work will help create the basis for subsequent research into specific diseases.”
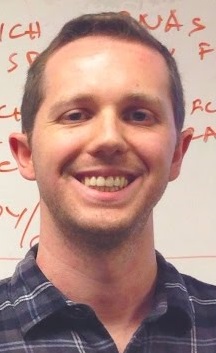
While pursuing the work, co-first author Dr. Vitor Onuchic was a graduate student in the BCM graduate program in quantitative and computational biosciences directed by Milosavljevic. The other co-first author, Eugene Lurie, is a graduate student in the BCM graduate program in molecular and human genetics.
Would you like to learn more about this work? Find it in the journal Science.
Other contributors to this work include Ivenise Carrero, Piotr Pawliczek, Ronak Y. Patel, Joel Rozowsky, Timur Galeev, Zhuoyi Huang, Robert C. Altshuler, Zhizhuo Zhang, R. Alan Harris, Cristian Coarfa, Lillian Ashmore, Jessica W. Bertol, Walid D. Fakhouri, Fuli Yu, Manolis Kellis, Mark Gerstein and the NIH Roadmap Epigenomics project. The authors are affiliated with one of the following institutions: Baylor College of Medicine, Yale University, Massachusetts Institute of Technology and the University of Texas Health Science Center at Houston.
Financial support for this project was provided by the Common Fund of the National Institutes of Health (Roadmap Epigenomics Program, grant U01 DA025956), the National Human Genome Research Institute (grants 5U24HG009446-02, U01 HG007610 and R01 HG008155), the National Institute of General Medical Sciences (grant GM122030-01) and the National Institute of Mental Health (grant R01 MH109978).