“Freeze-frame” synthetic proteins capture DNA events leading to cancer
Cancer scientists have focused on identifying signature genetic changes present in cancerous cells and understanding how these changes lead to cancer. Although this approach has delivered important advances, at Baylor College of Medicine Dr. Susan Rosenberg and colleagues wanted to get closer look at how these changes happen.
“In my lab we study how the genome – the genes in an organism – changes, in particular how the genome of normal cells changes to transform the cells into cancerous cells,” said Rosenberg, Ben F. Love Chair in Cancer Research and professor of molecular and human genetics, of molecular virology and microbiology and of biochemistry and molecular biology at Baylor. “But genomics, which allows us to compare the genes of normal cells with those of cancerous cells, is like reading the fossil record of these processes. We want to see how the real-time processes that change DNA happen, including all the intermediate steps.”
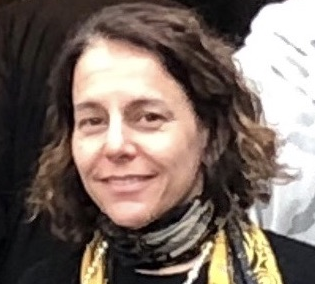
With this goal in mind, the researchers developed a new technology – synthetic proteins that allowed them to freeze in time and isolate ephemeral intermediate molecules that drive the genetic changes that transform normal cells into cancerous cells.
How cancer happens
Mutations in the cell’s DNA cause genetic changes that can steer a normal cell toward becoming cancerous and also cause resistance of cancers to chemotherapeutic drugs. Mutations result from many different sources, including imperfect DNA repair reactions and simple errors that occur when the cell is making copies of the DNA.
When cells divide and make copies of the instructions encoded in their DNA, the DNA unwinds and becomes vulnerable to damage that must be repaired. However, sometimes the process of repairing the DNA causes mutations and errors remain. When these errors accumulate over time, the cells may acquire characteristics of cancer.
“The process of editing the DNA is carried out by specific enzymes – proteins that work on DNA to fix the mistakes,” said Rosenberg, who also is leader of the Cancer Evolvability Program at the Dan L Duncan Comprehensive Cancer Center at Baylor.
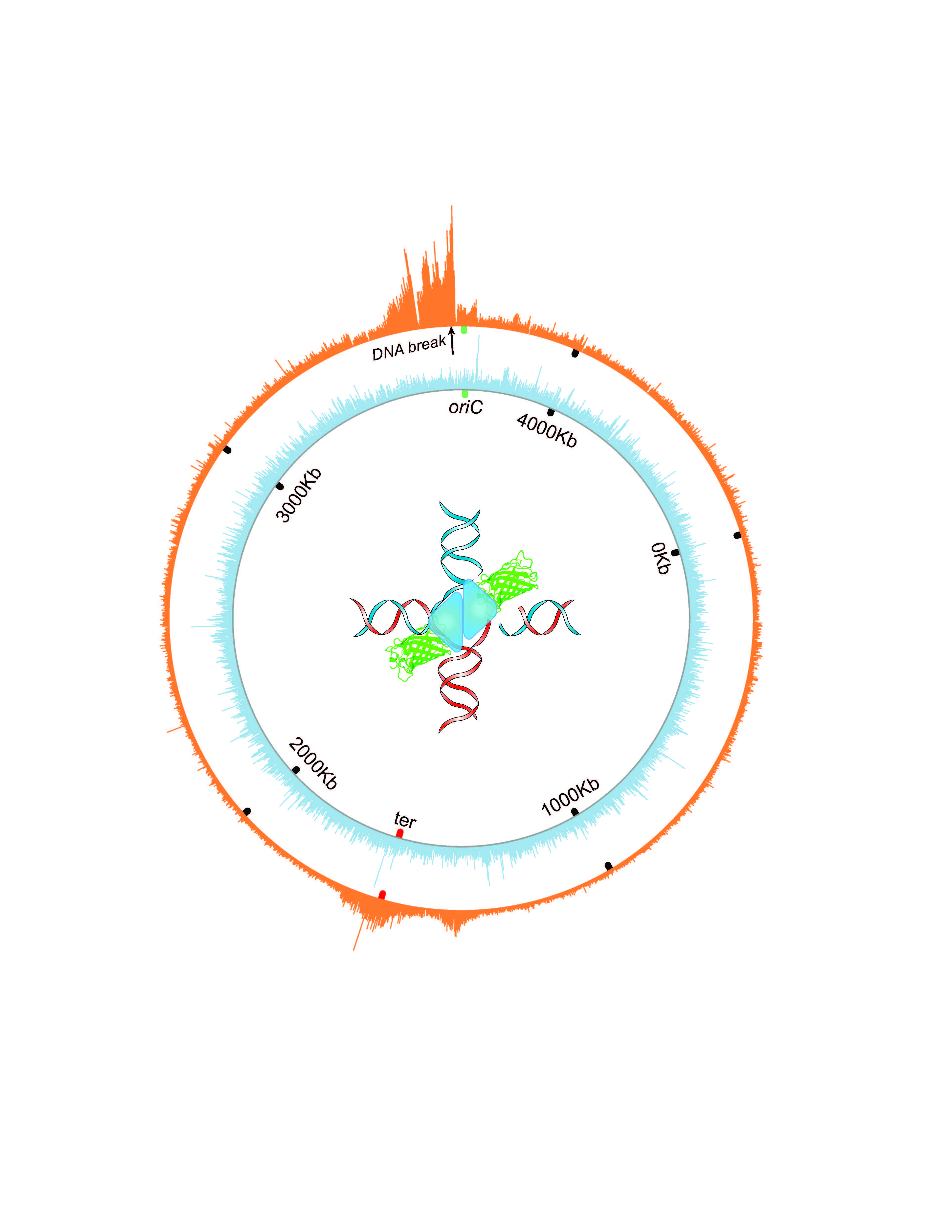
Editing DNA is a biochemical reaction in which an enzyme interacts with DNA that needs repair and promotes chemical changes that end up producing DNA without mistakes. DNA repair processes usually take several steps to be completed. Between the original DNA and the final product there are intermediate, short-lived DNA molecules. The intermediate molecules are crucial to the reaction, but are hard to study because they are transient and elusive. They are present for a fraction of a second as the enzyme catalyzes the changing of one molecule into another.
Freezing in time the elusive molecules that lead to cancer
“The intermediate molecules are the most important parts of biochemical reactions,” said Rosenberg. “They define what the reaction is and how it will proceed. But because they are transient and elusive, it’s really difficult to study them, especially in living cells. We wanted to do that. We decided to invent synthetic proteins that would trap DNA reaction intermediates in living cells.”
Other investigators also have attempted to trap intermediates, but they have only succeeded in a few biochemical reactions.
Rosenberg and colleagues carried out this study in the laboratory bacterium E. coli, which they and others have shown to be a reliable model of the genetic changes that occur in animal cells. In their paper, they discovered a new role of an E. coli protein related to five human cancer proteins. They then analyzed gene expression data from human cancers and were able to implicate two of the five E. coli-related human cancer proteins in potentially promoting cancer by a similar mechanism – one not previously implicated.
“The most exciting part in this paper for me is that we can learn something new about the mechanisms of cancer from the E.coli model,” said co-first author Qian Mei, who is a research assistant in the Rosenberg lab and a graduate student in the Systems, Synthetic and Physical Biology (SSPB) program at Rice University. “Even though bacteria and human cells are very different, many DNA repair proteins are highly conserved through evolution; this makes E. coli a good model to study how cells repair DNA or accumulate mutations.”
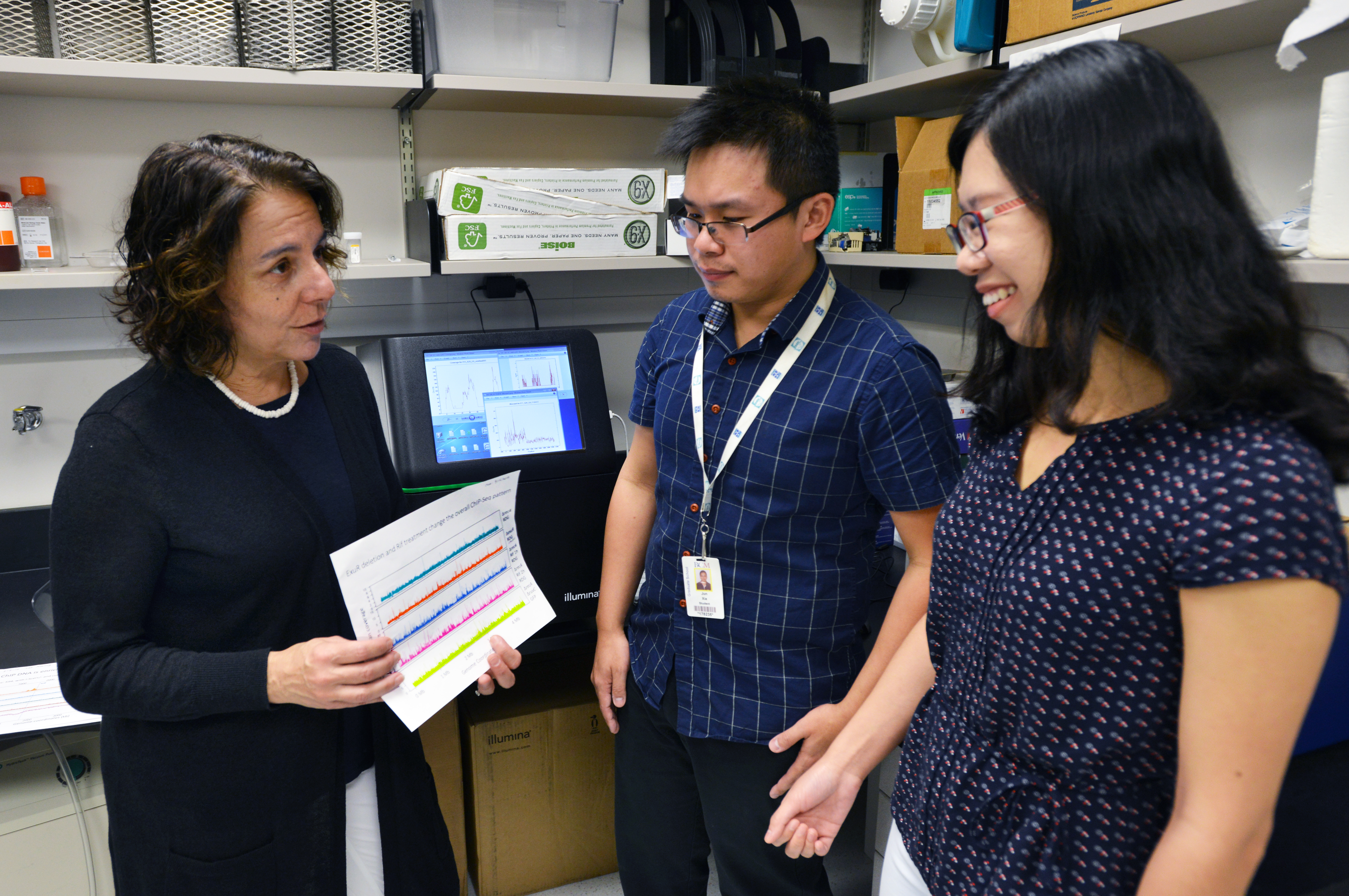
Rosenberg and colleagues think that their approach offers significant advantages. For instance, with the synthetic proteins, they have been able to identify specific DNA-repair intermediate molecules, their numbers in cells, rates of formation and locations in the genome and the molecular reactions in which they participate.
“It is most exciting that we are now able to trap, map and quantify transient DNA reaction intermediates in single living cells,” said co-first author Jun Xia, graduate student in the Rosenberg lab and in the Integrative Molecular and Biomedical Sciences program at Baylor. “This new technology helps us reveal the origins of genome instability.”
“When you know these reactions and the role each intermediate plays in the mechanisms that change DNA, you can think about making drugs that will stop them,” said Rosenberg. “In the future we hope we will be able to design drugs that target specific types of cancers; drugs that block the cells’ ability to evolve into cancer cells, instead of, or in addition to, traditional chemotherapies that kill or stop cancer cells from growing.”
Their results appear in Science Advances.
###
Other contributors to this work include Li-Tzu Chen, Chien-Hui Ma, Jennifer A. Halliday, Hsin-Yu Lin, David Magnan, John P. Pribis, Devon M. Fitzgerald, Holly M. Hamilton, Megan Richters, Ralf B. Nehring, Xi Shen, Lei Li, David Bates, P.J. Hastings, Christophe Herman and Makkuni Jayaram. The authors are affiliated with one or more of the following institutions: Baylor College of Medicine, Rice University, the University of Texas at Austin and the University of Texas MD Anderson Cancer Center.
This work was supported by a gift from the WM Keck Foundation, and its early stages were supported by a National Institutes of Health Director’s Pioneer Award DP1-CA174424 to Rosenberg. The work also was supported by the National Aeronautics and Space Administration through the NASA Astrobiology Institute under Cooperative Agreement No. NNA13AA91A issued though the Science Mission Directorate, Cancer Prevention and Research Institute of Texas (CPRIT) RP140553, and CPRIT Baylor College of Medicine Comprehensive Cancer Training Program Postdoctoral Fellowship RP160283, NIH R01 grants CA190635, GM102679, GM106373, GM88653, National Science Foundation grant MCB1049925, Robert F Welch Foundation Award F-1274, and the Baylor College of Medicine (BCM) Cytometry and Cell Sorting Core with funding from the NIH (P30-AI036211, P30-CA125123, and S10-RR024574), and the BCM Integrated Microscopy Core with funding from the NIH (HD007495, DK56338, and CA125123) and CPRIT RP150578, the Dan L. Duncan Comprehensive Cancer Center and the John S. Dunn Gulf Coast Consortium for Chemical Genomics.